
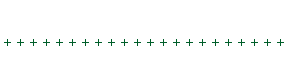

Summary of cell communication techniques
All communication involving cells can be explained in terms of a force of attraction (called affinity) between molecules. Components that allow detection are called receptors and they often have a cavity shape which allows other molecules to lock into them. The receptor has an affinity for a particular message or signal.
Receptor molecules can migrate to the surface of a cell. They will have a complementary shape to molecules on the outside of the cell which lock into them. In this way cells can communicate with each other
Affinities have strength values depending upon the exactness of molecular shape fits. A probability constant determines the chance of a connection - called an association constant. Similarly there is a disassociation constant, which determines the probability of breaking away. This in turn gives another factor called the velocity of separation of the two units.
Affine (highly affinitive) connections are slow for purposes of communication. For some fast signalling purposes (rapid information of the order of one thousandth of a second), strong disassociation constants - weak affinities - are necessary to achieve higher speed. Many molecules can vary the shape of their receptors to change transmission speed and may use this to get rid of signals as soon as they have been registered. Released signal molecules may also have to be destroyed sometimes.
Receptors can be quite complicated structures with atomic weights running at 10000 daltons (100 amino acid sequences). Shapes of proteins are difficult to observe although they can be modeled to some extent by computer. Some messages consist of simple amino acids which have been modified simply by using an enzyme to add or remove an atom or two.
Although the diagrams above have shown only proteins acting upon other proteins, some protein effectors can regulate pumps and channels at the surface of cells. The pumps and channels can regulate the entry of ions of various chemicals substances such as sodium, potassium, chlorine or calcium into or out of a cell. These will have a chemical effect on other proteins in the cell as an indirect action of a protein processing mechanism. Effectors can often do their job using slight modifications such as adding a phosphate group to a protein to radically change its shape or effect on a processing arrangement.
Molecules can have address labels attached to them by the action of proteins (usually by way of adding sugars). This allows proteins to be channeled around a cell in micro tubules which act much the same way as an underground railway in a large city.
Communication molecules can be hard to detect because often they are quite small: some are of the order of one billionth of a gram and have to be separated out from other molecules in the cell before they can be studied. Many cells communicate by using cell adhesion molecules (CAMs). These consist of a short sequence of four amino acids arginine (R), glycine (G), asparine (N) and serine (S) with a mirror sequence (RGNS/SNGR) serving as the receptor. Many cells have auto-receptors i.e. receptive to their own cell signals. They can be used in regulatory feedback loops. Cells and proteins can also capture protein messages and signals to be able to reuse them.
Generally, we can think of chemical signals as being stimulatory - or inhibitory - for controlling levels of activity. However, the situation is seldom this simple as there are hundreds of different types of signals and they all work in concerted coordination to carefully regulate what happens in a cell according to a wide range of influencing factors. Cell processes are not so much on or off, but, held in a dynamic state of tension. Many different protein messages or components may determine the state of a cell (for example, the cell responsible for secreting a pituitary hormone - prolactin - has been shown to respond to at least twenty different signals).
Signaling molecules are not necessarily exclusive to any one particular cell type or any one special process. The same message signals can be used for different purposes at different locations. The specialization of signals is influenced by how long they can last without disintegrating - i.e. sensitivity to degradation mechanisms. This also influences whether cells can be used for short distance or long distance communications. Proteins that are fairly stable are used to communicate over large distances between cells. Proteins that break up easily are used for short local communications.
The ability of cells and proteins to recognize and respond to messages is a function of how easily they can match affinities in a sea of noise. This ability can be reduced or enhanced as a control function. The mystery is not how cells address messages to each other, but, how they can extract messages from a myriad of other molecules which may be in suspension.
Coupled proteins can act like railway switches to re- route messages. They can also act like read heads to take information from a signal. Sometimes the action of an effector can modify its own receptor to provide negative or positive feedback or change the sensitivity.
Signals have various levels of organization such that one set of signals take precedence in one situation and another set take precedence in another. This is used in such reactions where the sudden appearance of danger can heighten flight or fight responses and suspend or modify normal body regulation patterns. Some signals, such as stress hormones, can be slow acting and long lasting. Others, such as those that provide surges of adrenalin in time of danger, can be fast acting.
Nerve cells, glandular cells and lymphocytes are able to express and receive common signals. This allows a communication system to be established between the brain and the immune system. If neurotransmitters can be recognized by a lymphocyte and if a neurone can decipher immune response messages the brain can direct the body to start healing itself. Lymphocytes are capable of producing certain stress hormone so in cases of illness the immune system can bypass the neurological controls that normally regulate the bodily system and functions.
Reflecting on how these signaling systems could have evolved, enzymes are in effect a recognition system and could have created initial crude systems of control. The receptor/signal pair could have evolved from an enzyme/substrate system. In this evolutionary process the role and complexity of the receptors and their systems could have grown increasingly more complex.
All the different components of these receptor complexes could have evolved as a sequence of random events that change nucleotide sequences around or connected them with different transcription factors. This view sees the coupling structures and their assemblies as Lego like components, which evolution can manipulate into vast numbers of possible variations.
This modular view of biological systems sees organization proceeding in a hierarchical form and with changes possible at all levels in the hierarchy evolution can proceed in a multitude of different ways and directions.
The intricate organization and the complexity of biological systems often seem beyond rational explanation. Yet, it has been found that even simple rules of diversification can lead to an enormous repertoire of message signaling. Even an elementary membrane protein of yeast cells can produce a very complex communication system based upon simple rules applicable to diversification. Using various combinations of proteins to open and close channels through cell membranes messages can be directed around the cell to appropriate receptors. Enzymes can also be directed by effectors to separate out components of signals and messages, or, join them together to effect more complex action.
The evolutionary process that creates complex systems also builds in many duplicates and backups. The more important or vital the function of a cell complex the more redundancy of signaling is built into the system. Maybe dozens of message sources providing a similar message via various routes can act concurrently to achieve similar effects. This allows faults and breakdowns to be tolerated and ensures that a single error does not disrupt the system (much like an aeroplane might have several independent auto control systems in operation at the same time to allow for component failure).
Some cells, neurones, establish permanent physical connections with others so that they can form networks of inter cell communications. These cells can route information around the network by controlling the flow of protein messengers at the point of their connections (synapses). It has been shown that neurone cells in the brain are not randomly connected but are routed according to marker patterns dictated by the interaction of proteins. These proteins, specified by nucleotide sequences in the DNA of the genome, obey simple physical laws yet their overall effect is to form the complexity of the structure we call the brain.
The growth of brain neurone cells follow precise signaling rules, axons and dendrites grow out of the main cell bodies according to the controlled production of a host of different hormones. The proportions of these hormones determine the rate of growth as different neurones compete with each other for the same connection sites. . This same technique of selective mixes and combinations of hormones also determine the type of synapse. . These mixes in turn are controlled by the interactions of the proteins that have been predetermined by the sequence of nucleotides on the genome.
Despite the laying down of specific pathways and synaptic connections by the DNA this is far from being a rigid system because protein messages, coming from sense organs all over the body, can interact with these processes. Logic gates and receptor/effector combinations can produce any variety of hormones, enzymes, peptides or other proteins. These can be labeled and addressed, to be sent anywhere in the body. This allows genetic information and information stored in the brain to be modified by the environment.
To make sure that protein messengers prompted by environmental stimuli do not totally disrupt or swamp the neural system there is a Hierarchical order of authority. This often gives preference to messages emanating from the genome as a result of the evolutionary process. This can be thought of as acting like a moderated list serve where subscribers can vary their topics of conversation only within a specified range tolerated by the list moderator.
The intricate hierarchical systems of interactive control mechanisms that have evolved in the designs of biological life forms defy the imagination. They seem far too sophisticated to have evolved by chance in a blind evolutionary process. Yet, as we shall see later, such systems can evolve by chance from simple beginnings.
If it were possible to trace the processes of complexity coming into existence step by step, every bit of the way would seem simple and obvious, but, to try to look back into a system which has already evolved is quite impossible. This is somewhat similar to the problem we encountered earlier when trying to work backwards from the analog shape of a protein to the binary sequence which specified it. The observed solution is a solution to only one of billions of possible problems. Going forward in this type of processing is far easier than trying to work backwards.
Although cells take their instructions from a genome that is common to all cells, there are special sections on the genome which can be variable or different in every cell. These variable sectors allow different proteins to be created from the same signal.
End of article